Pictures
Long-Axis Rotation
A missing degree of freedom in avian bipedal locomotion
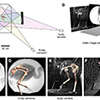
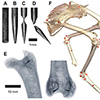
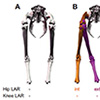
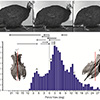
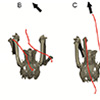
Birth of a Dinosaur Footprint
Subsurface 3D motion reconstruction and discrete element simulation reveal track ontogeny
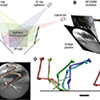
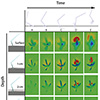
Fish Suction Power
Swimming muscles power suction feeding in largemouth bass
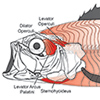
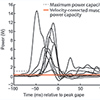
Fish Bite Force
Gape-specific bite force and prey-size specific predator performance in
the snail-eating black carp
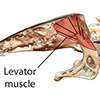
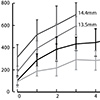
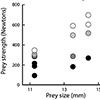
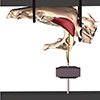
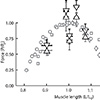
Jump Cut
Biomechanics of male and female ACL-intact and ACL-reconstructed
athletes during a jump-cut maneuver
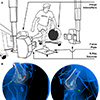
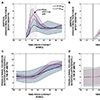
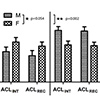
Soft Tissue Artifact
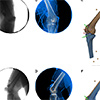
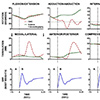
Fish Feeding
Biomechanics of jaw protrusion in common carp
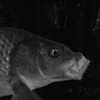
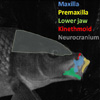
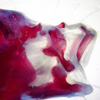
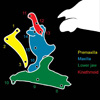
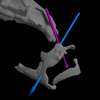
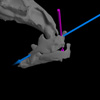
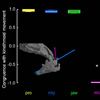
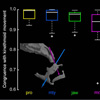
Iguana Breathing
Rib kinematics & intercostal muscle strain during breathing





Pig Feeding
Marker-based XROMM analysis of mastication in minipigs
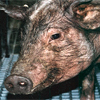
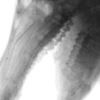
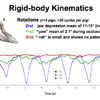
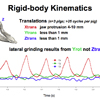
Duck Feeding
Kinematics of the quadrate bone during feeding in mallard ducks
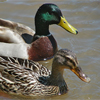
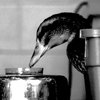
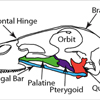
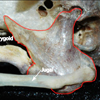
Hardware
Mobile C-arm fluoroscopes and biplanar x-ray rooms
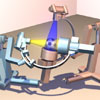
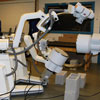
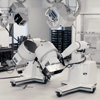
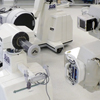